Moles and Molar Mass: The Superpower for Counting in the Microscopic World - AP Chemistry Complete Notes
Welcome, AP Chemistry students, to the fascinating world of moles and molar mass! This unit is the foundation for understanding how we work with chemicals in the real world. Imagine trying to cook a recipe by counting individual grains of rice or flakes of salt – that’s what chemistry would be like without moles! Today, we’ll unpack these concepts and unlock the ability to work with vast numbers of atoms and molecules with ease.
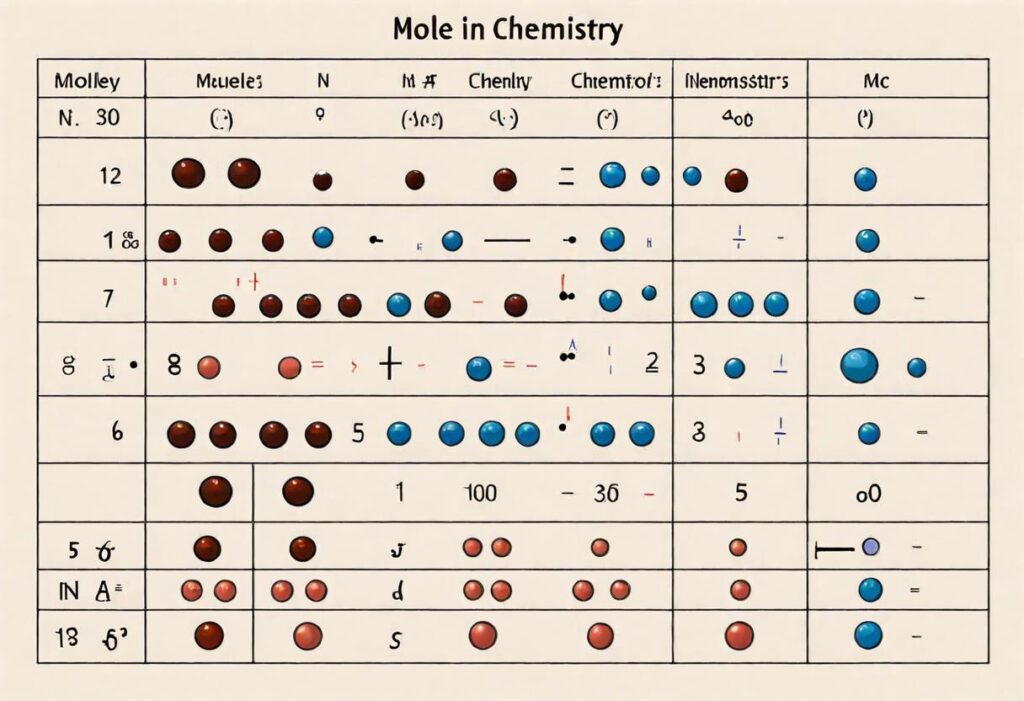
The Challenge: Counting the Uncountable
Chemistry deals with the microscopic world of atoms and molecules. These particles are incredibly tiny – a single water molecule (H2O) is about 0.000 000 000 03 meters wide! Counting them individually is simply impossible. So, chemists needed a clever way to work with these immense quantities.
Enter the Mole: Our Super-Sized Unit
Think of a dozen eggs. It’s a convenient way to buy eggs without counting each one. Similarly, a mole (abbreviated mol) is a unit that represents a specific number of atoms or molecules – a whopping 6.022 x 10^23 (six hundred two sextillion)! This number, known as Avogadro’s number, is a constant that acts as a conversion factor between the microscopic and macroscopic world.
Molar Mass: The Mass of a Mole
Now, imagine a dozen eggs all weigh exactly the same. Molar mass is like that, but for atoms or molecules. It’s the mass of one mole of a substance expressed in grams (g/mol). Here’s the key connection: the molar mass of an element (like sodium, Na) is numerically equal to its atomic mass (found on the periodic table) in grams per mole. It’s like a unique fingerprint that tells you the mass of a giant collection (one mole) of those tiny atoms.
Calculating Molar Mass: A Piece of Cake (Almost!)
For molecules (compounds made from two or more elements), calculating molar mass involves some simple math. Here’s the recipe:
- Identify the elements present in the molecule.
- Look up the atomic mass of each element from the periodic table (pay attention to units – they should be in amu, atomic mass units).
- Multiply the atomic mass of each element by the number of atoms of that element in the molecule (according to the chemical formula).
- Add up the products from step 3.
Voila! You’ve just calculated the molar mass of your molecule. Let’s see this in action:
Example: Water (H2O)
- Elements: Hydrogen (H) and Oxygen (O)
- Atomic masses: H = 1.008 amu, O = 15.999 amu
- Calculations:
- H: 1.008 amu x 2 atoms = 2.016 amu
- O: 15.999 amu x 1 atom = 15.999 amu
- Molar mass of H2O = 2.016 amu + 15.999 amu = 18.015 amu (remember, molar mass is in g/mol, so we’ll convert amu to grams later)
The Power of Moles and Molar Mass
So, why are moles and molar mass so important? Here are some superpowers they unlock:
- Converting Between Mass and Number of Particles: We can use the molar mass as a conversion factor to find the number of atoms or molecules in a given mass of a substance (and vice versa). Imagine having 18.015 grams of water. How many water molecules is that? We can use the molar mass of water (18.015 g/mol) to convert grams to moles and then multiply by Avogadro’s number to get the number of molecules.
- Stoichiometry Made Easy: Chemical reactions involve specific ratios of reactants and products. Moles allow us to express these ratios in a meaningful way, making it easier to calculate quantities needed or produced in a reaction.
- Concentration Units: Molarity (M), a common concentration unit, is expressed in moles per liter (mol/L). Knowing the molar mass lets us convert between the mass of a solute (dissolved substance) and its concentration in solution.
Moles and Molar Mass: Advanced Concepts (For the Eager AP Chemistry Student)
We’ve covered the fundamental aspects of moles and molar mass. Now, let’s delve deeper into some advanced concepts you might encounter in AP Chemistry:
1. Molar Masses of Ions and Polyatomic Ions:
- Ions (charged atoms) and polyatomic ions (covalently bonded groups of atoms that act as a single unit) also have molar masses.
- To find the molar mass of an ion, simply add the atomic masses of its constituent elements, considering their charges.
- For polyatomic ions, the molar mass is calculated like a molecule, using the atomic masses of the elements involved.
Example: Sodium Sulfate (Na2SO4):
- Na2SO4 consists of 2 sodium (Na+) ions and a sulfate (SO4²⁻) polyatomic ion.
- Molar mass of Na: 22.989 g/mol (from periodic table)
- Molar mass of SO4²⁻: (1 x 32.065 g/mol for S) + (4 x 15.999 g/mol for O) + (2 x -1.008 g/mol for the negative charges) = 96.062 g/mol
- Total molar mass of Na2SO4: (2 x 22.989 g/mol for Na) + 96.062 g/mol for SO4²⁻ = 142.04 g/mol
2. Empirical and Molecular Formulas:
- The empirical formula gives the simplest whole-number ratio of atoms in a compound.
- The molecular formula shows the actual number of atoms in a molecule.
- Molar mass can help differentiate between empirical and molecular formulas.
- If the calculated molar mass based on the empirical formula is a whole-number multiple of the experimentally determined molar mass, then the empirical formula is likely the molecular formula as well.
Example:
An unknown compound is found to have an empirical formula of CH2O and an experimental molar mass of 60 g/mol.
- Molar mass based on CH2O: (1 x 12.011 g/mol for C) + (2 x 1.008 g/mol for H) + (1 x 15.999 g/mol for O) = 30.016 g/mol
- Since the experimental molar mass (60 g/mol) is double the calculated molar mass (30.016 g/mol), the molecular formula is likely C2H4O2.
3. Limiting Reagents and Percent Yield:
- In chemical reactions, the reactant present in the limiting amount (limiting reagent) dictates how much product can be formed.
- Moles and molar masses are crucial for calculating the amount of product formed based on the limiting reagent and the percent yield (the actual amount of product obtained compared to the theoretical amount).
4. Ideal Gas Laws and Molarity:
- Ideal gas laws relate pressure, volume, temperature, and amount (number of moles) of a gas.
- Molarity (concentration of a solute in moles per liter of solution) is often used in conjunction with ideal gas laws to solve problems involving gases.
Beyond the Textbook: Sharpening Your Skills
- Practice, Practice, Practice: The best way to master moles and molar mass is to solve numerous practice problems. Focus on different types of calculations – converting between mass and moles, using molar mass in stoichiometry, and applying concepts like limiting reagents and ideal gas laws.
- Unit Analysis: Get comfortable with unit conversions. Molar mass bridges the gap between grams and moles, allowing you to work with both macroscopic and microscopic quantities. Pay attention to units throughout your calculations.
- Dimensional Analysis: This problem-solving technique helps you set up calculations logically. By writing down units along with quantities, you can ensure you’re on the right track and arrive at the correct answer with the appropriate units.
The Takeaway
Moles and molar mass are powerful tools that unlock the secrets of the microscopic world in chemistry. By understanding these concepts and practicing their application, you’ll be well-equipped to tackle various problems in AP Chemistry and beyond. Remember, the key is to build a solid foundation, practice consistently, and don’t hesitate to seek help if needed. With dedication and a little perseverance, you’ll master these concepts and conquer the wonders of chemistry at the atomic and molecular level!
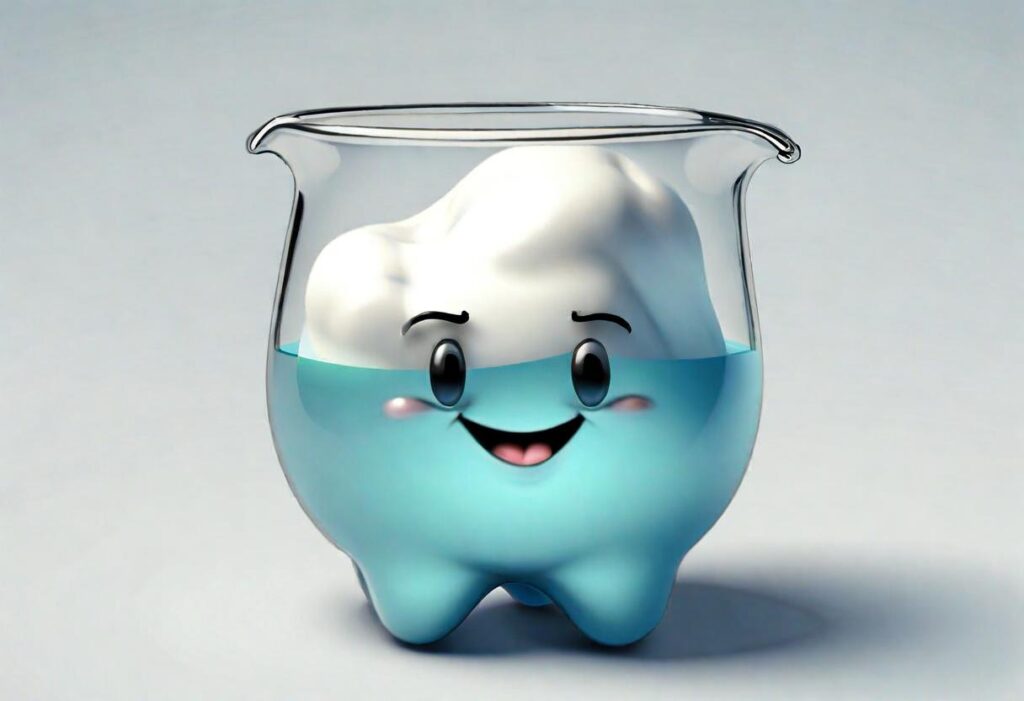
Moles and Molar Mass: Real-World Applications (A Glimpse into the Power of Tiny Quantities)
So far, we’ve explored moles and molar mass in the context of AP Chemistry. But did you know these concepts have far-reaching applications in the real world? Let’s delve into some fascinating examples:
1. Medicine and Pharmaceuticals:
- Drugs are often administered in specific dosages. Molar mass helps convert between the prescribed milligram (mg) or microgram (μg) dose of a drug and the number of molecules a patient needs to receive for effective treatment.
- Understanding molar mass is crucial for developing new drugs. Chemists can calculate the amount of a potential drug molecule needed for clinical trials or large-scale production.
2. Materials Science and Nanotechnology:
- Molar mass plays a vital role in materials science, where scientists work with specific quantities of atoms to create new materials with desired properties.
- In nanotechnology, where materials are manipulated at the atomic and molecular level, molar mass allows researchers to precisely control the number of atoms or molecules used to build nanostructures.
3. Environmental Science and Sustainability:
- Molar mass is used to measure the concentration of pollutants in air and water. By analyzing the number of moles of a pollutant per liter of water (or per cubic meter of air), scientists can assess environmental health and develop strategies for remediation.
- Molar mass calculations are also crucial in developing sustainable technologies, such as using specific amounts of catalysts to optimize chemical reactions that minimize waste production.
4. Food Science and Nutrition:
- Molar mass helps determine the number of moles of nutrients (like vitamins and minerals) present in a specific serving of food. This information is crucial for labeling food products and ensuring consumers receive the recommended daily intake of essential nutrients.
- Molar mass calculations are also used in food processing to control the amount of additives or preservatives used in food products, ensuring safety and quality control.
5. Energy Production and Storage:
- In battery technology, molar mass helps determine the amount of electrode material needed for efficient energy storage and delivery.
- Molar mass calculations are also used in nuclear power plants, where the number of moles of fissile isotopes (atoms that readily undergo nuclear fission) determines the amount of energy released in a nuclear reaction.
The Final Word: A Microscopic World with Macroscopic Impact
As you can see, moles and molar mass are not just abstract chemical concepts. They have a profound impact on various fields, from medicine and materials science to environmental sustainability and energy production. By understanding these powerful tools, you gain a deeper appreciation for the intricate workings of the world around us, from the food we eat to the technologies we rely on.
Remember: The journey of scientific discovery often starts with understanding the fundamental building blocks – atoms and molecules. Moles and molar mass provide the key to unlocking the secrets of these tiny particles and their immense impact on our macroscopic world. Keep exploring, keep learning, and never underestimate the power of the microscopic!
Practice Question – AP Chemistry : Moles and Molar Mass
Question 1:
What is the molar mass of sodium chloride (NaCl)?
Solution:
- Look up the atomic masses: Na = 22.989 g/mol, Cl = 35.453 g/mol
- Molar mass of NaCl = 22.989 g/mol (Na) + 35.453 g/mol (Cl) = 58.442 g/mol
Question 2:
If you have 3.00 g of calcium carbonate (CaCO3), how many molecules of CaCO3 are there?
Solution:
- Find the molar mass of CaCO3: Ca = 40.078 g/mol, C = 12.011 g/mol, O = 15.999 g/mol (x3) Molar mass of CaCO3 = 40.078 g/mol + 12.011 g/mol + (15.999 g/mol x 3) = 100.09 g/mol
- Convert grams to moles: 3.00 g / 100.09 g/mol = 0.0300 mol CaCO3
- Use Avogadro’s number (6.022 x 10^23) to convert moles to molecules: 0.0300 mol x 6.022 x 10^23 molecules/mol = 1.81 x 10^22 molecules CaCO3
Question 3:
In the reaction 2H2 + O2 -> 2H2O, how many moles of water (H2O) are formed when 4.00 moles of hydrogen (H2) react?
Solution:
- Look at the stoichiometric coefficients: 2 moles H2 produce 2 moles H2O
- Since we have 4.00 moles of H2, and the ratio is 2:2, the number of moles of H2O formed is also 4.00 moles.
Question 4:
A solution is prepared by dissolving 10.0 g of potassium nitrate (KNO3) in enough water to make 250 mL of solution. What is the concentration of the solution in molarity (M)?
Solution:
- Find the molar mass of KNO3: K = 39.098 g/mol, N = 14.007 g/mol, O = 15.999 g/mol (x3) Molar mass of KNO3 = 39.098 g/mol + 14.007 g/mol + (15.999 g/mol x 3) = 101.1 g/mol
- Convert grams of KNO3 to moles: 10.0 g / 101.1 g/mol = 0.0989 mol KNO3
- Calculate the concentration (M): 0.0989 mol / 0.250 L = 0.396 M KNO3
Question 5:
An ideal gas occupies 5.00 L at a pressure of 1.00 atm and a temperature of 25°C. If the gas is helium (He), how many moles of He are present?
Solution:
(We can use the ideal gas law PV = nRT, but this question can also be solved using unit conversion with molar mass if you’re comfortable assuming ideal gas behavior).
- Find the molar mass of He: 4.003 g/mol
- Given the volume (L) and pressure (atm), we can convert these units to those required for the ideal gas constant R (usually 0.08206 L atm/mol K). You’ll need to find the conversion factors yourself based on the units provided for R.
- Once you have all the units consistent, rearrange the ideal gas law to solve for moles (n) of He.
Moles and Molar Mass: Frequently Asked Questions (For the Curious Chemist)
By now, you’ve gained a solid understanding of moles and molar mass. But you might still have lingering questions. Here are some commonly asked questions to solidify your knowledge:
1. What if the atomic mass on the periodic table isn’t a whole number?
The periodic table often lists atomic masses as average values because elements exist in multiple isotopes (atoms with the same number of protons but different numbers of neutrons). When calculating molar mass, use the average atomic mass provided.
2. How do I handle units when working with molar mass?
Molar mass is expressed in grams per mole (g/mol). Ensure all your quantities (mass, number of atoms/molecules) have compatible units before using the molar mass in calculations.
3. Can moles be used for units other than atoms and molecules?
Yes! Moles can be used for any collection of particles. For example, we can talk about moles of electrons or photons. However, the concept of molar mass is specific to atoms and molecules.
4. What are limitations of using the ideal gas law with molar mass?
The ideal gas law assumes perfect gas behavior, which isn’t entirely true for real gases. Molar mass works well for ideal gases at low pressures and high temperatures. For real gases at high pressures or low temperatures, deviations from ideal behavior need to be considered.
5. How do moles relate to concentrations like parts per million (ppm)?
Molarity (mol/L) is the most common concentration unit used with moles. ppm (and similar units like ppb – parts per billion) express concentration as a ratio of parts of a solute to millions (or billions) of parts of the solution. While not directly related to moles, you can convert between molarity and ppm if you know the total volume of the solution and the molar mass of the solute.
6. Are there any online resources for practice problems?
Absolutely! Websites and textbooks offer practice problems on moles and molar mass. Utilize online resources like Chemistry Bench AP Chemistry Classes and Blogs or practice problems from past AP Chemistry exams to test your understanding.
The Final Thought
Learning chemistry can be like exploring a new world. Moles and molar mass are your compass and map, helping you navigate the vast and fascinating realm of atoms and molecules. Remember, the key to success is consistent practice, a willingness to ask questions, and a passion for discovery. So, keep exploring, keep asking “why” and “how,” and never stop unraveling the wonders of the microscopic world!